At WiseGEEK, we're committed to delivering accurate, trustworthy information. Our expert-authored content is rigorously fact-checked and sourced from credible authorities. Discover how we uphold the highest standards in providing you with reliable knowledge.
What are Some Unusual Forms of Space Propulsion?
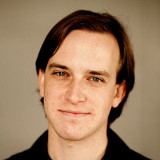
Typical forms of space propulsion today are solid rocket boosters, liquid rockets, and hybrid rockets. All carry their fuel on board and use chemical energy to produce thrust. Unfortunately, they can be very expensive: it may take 25-200 kilograms of rocket to deliver a 1-kg payload to low Earth orbit. Lifting a kg to low Earth orbit costs a minimum of $4,000 US Dollars (USD), as of 2008. $10,000 USD may be more typical.
The chemical rocket approach to space launch and travel is fundamentally limited. Because a rocket must propel its own fuel upwards through the densest part of the atmosphere, it isn't very cost effective. A more recent invention is the private spacecraft SpaceShipOne, which used a carrier craft (White Knight) to carry it to 14 km (8.7 mi) altitude before launch. At this height, greater in altitude than Mt. Everest, SpaceShipOne is already above 90% of the atmosphere, and is able to use its small hybrid engine to travel the rest of the way to the edge of space (100 km altitude). Early, cheap, reusable tourist spacecraft are likely to be based on this model.
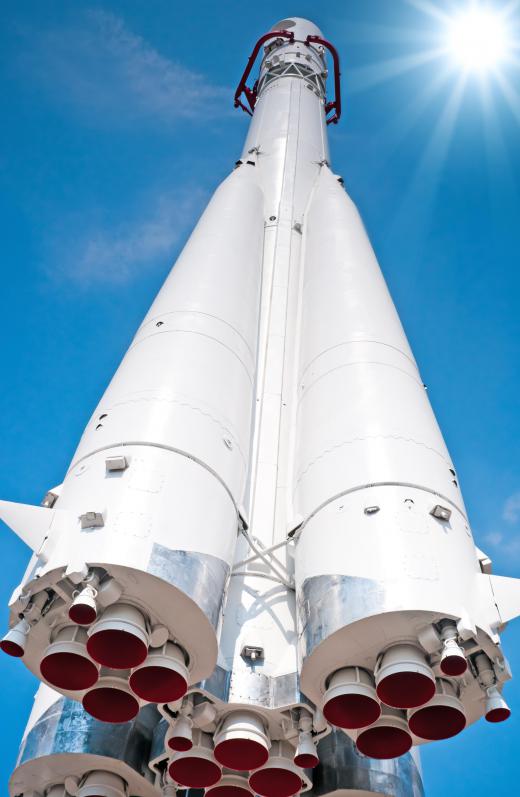
Beyond the chemical rocket paradigm, there are several other forms of space propulsion that have been analyzed. Ion thrusters, in particular, have already been used successfully by several spacecraft, including Deep Space 1, which visited the comet Borrelly and asteroid Braille in 2001. Ion thrusters operate like a particle accelerator, throwing ions out the back of the engine using an electromagnetic field. For longer trips, such as from the Earth to Mars, ion thrusters offer better performance than conventional forms of space propulsion, but only by a small margin.
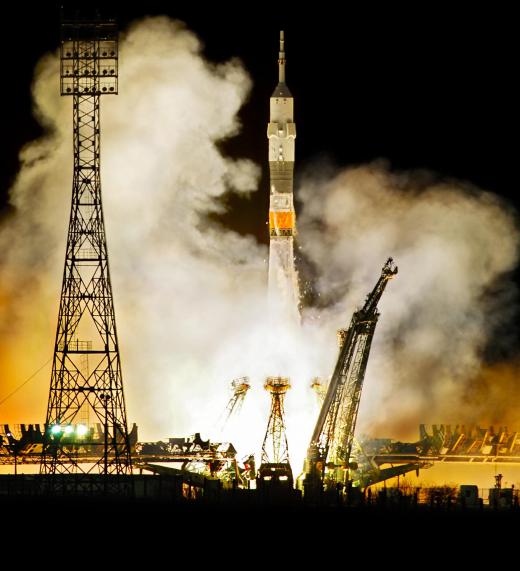
More advanced forms of space propulsion include nuclear pulse propulsion and other nuclear-powered approaches. The power density of a nuclear power plant or nuclear bomb is many times greater than that of any chemical source, and nuclear rockets would be correspondingly more effective. Nuclear pulse propulsion that one reference design from the 1960s, called Orion — not to be confused with the Orion Crew Exploration Vehicle of the 2000s — that it could deliver a 200-person crew to Mars and back in only four weeks, compared to 12 months for NASA's current chemically-powered reference mission, or Saturn's moons in seven months.
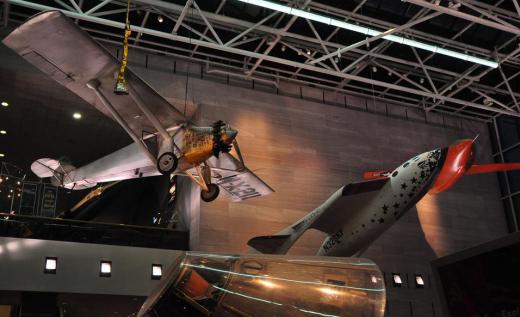
Another design called Project Daedalus would have required only about 50 years to make it to Bernard's Star, 6 light-years away, but would require some technological progress in the area of inertial confinement fusion (ICF). Most research on nuclear pulse propulsion was canceled due to the Partial Test Ban Treaty in 1965, although the idea has received renewed attention as of late.
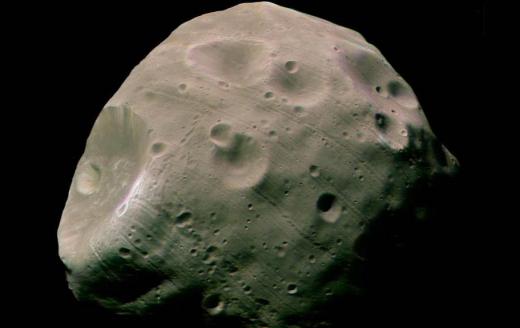
Another form of space propulsion, solar sails, were examined in some detail in the 1980s and 1990s. Solar sails would use a reflective sail to accelerate the payload using the radiation pressure of the Sun. Carrying no reaction mass, solar sails could be ideal for fast travel away from the Sun. Although solar sails may take weeks or months to accelerate to an appreciable speed, this process could be leapfrogged by using Earth or space-based lasers to direct radiation onto the sail. Unfortunately, the technology for folding and unfolding an extremely thin solar sail is not yet available, so construction may have to occur in space, complicating matters considerably.
Another, more futuristic form of space propulsion would be to use antimatter as a fuel for propulsion, like some spaceships in science fiction. Today, antimatter is the most expensive substance on Earth, costing about $300 billion US Dollars per milligram. Only several nanograms of antimatter have been produced thus far, about enough to illuminate a light bulb for several minutes.
The key distinction between many of the mentioned technologies and chemical rockets is that these technologies may be able to accelerate spacecraft to near-light speeds, while chemical rockets cannot. Thus, the long-term future of space travel lies in one of these technologies.
AS FEATURED ON:
AS FEATURED ON:


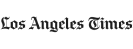

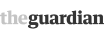
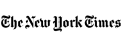
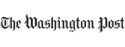


Discuss this Article
Post your comments